Green Energy
Geothermal Energy
The Earth as an Energy Source
The geothermal energy available from the Earth is potentially enormous. A United States Government energy agency estimates that the total energy available from global geothermal resources is approximately 15,000 times the energy contained in all the known oil and gas reserves in the world. Unlike solar and wind energy, the supply of geothermal energy is constant and doesn't vary with the time of day or change with the weather. Although geothermal energy may always be available when it is needed, like the other two sources it is not always availablewhere it is needed.
The Earth's core maintains temperatures in excess of 6000°K due to the heat generated by the gradual radioactive decay of the elements it contains. Modern estimates (Sclater 1981) for the total present rate of radioactive heat generation within the Earth are about 2 × 1013 W. This heat energy continuously flows outwards from the hot core due to conductive and convective flows of the molten mantle beneath the crust.
Estimates of the mean heat flux through the Earth's surface resulting from its radioactive core vary between 0.04 and 0.08 Watts per square meter. At the surface the heat dissipates into the atmosphere and space. This geothermal heat flow is trivial compared with the 1000 W/m2 of solar energy impinging on the surface of the Earth in the other direction from the Sun (1367 W/m2 at the outer surface of the atmosphere). Never the less it is sufficient to allow harvesting of geothermal energy on a commercial basis.
The diagram below shows the Earth's temperatures resulting from its internal heat generation and heat flows. The section on Solar Power describes the solar energy flows coming from external sources.
The Earth's Layers
- Inner Core - The inner core is solid with a radius of about 1,220 km and consists of about 80% Iron and 5% to 10% Nickel, with a temperature of up to about 7,200°K.
- Outer Core- The outer core, also mainly Iron and Nickel, is in a liquid state and is about 2,260 km thick. Melted rock is also called Magma
- Gutenberg Discontinuity Marks the boundary between the outer core and the inner mantle.
- Mantle is about 2900 kms thick surrounding the core and contains 83% of the volume and most of the mass of the Earth.
- Lower (Inner) Mantle (semi-rigid) - The deepest parts of the mantle, just above the core.
- Upper (Outer) Mantle is about 670 kms thick with two distinct regions, the hotter innermost part is plastic (flowing) while the cooler outermost part is rigid.
- Upper Mantle (flowing) = Asthenosphere - The innermost part of the upper mantle exhibits plastic (flowing) properties. It is located below the rigid lithosphere and is between about 100 and 250 km thick starting about 100-200 kms below the Earth's surface and possibly extending to a depth of 400 kms.
- Upper Mantle (rigid) - The rocky uppermost part of the mantle is part of the lithosphere.
- Lithosphere - The lithosphere is defined as the solid rocky region about 100-200 km thick which spans the crust and the rigid upper mantle.
- Mohorovicic (Moho) Discontinuity - is the boundary between the Earth's crust and the upper mantle.
- Crust - The Earth's crust occupies just 1% of the Earth's volume with a thickness averaging just 15 km. In scale size, this is only one fifth of the thickness of a typical egg shell. The temperature at the Earth's surface is typically 25 °C (298°K)
- Continental Crust - the exposed thick parts of the Earth's crust, (not located under the ocean). The average continental crust thickness is 35 km. The maximum thickness is 90 km below Himalayas and the minimum is 25 km at its thinnest in various places.
- Oceanic Crust - The part of the Earth's crust located under the oceans is thinner, only about 5 to 11 km thick.
- Ocean - large bodies of water up to 3.7 km deep sitting on top of the oceanic crust. The water temperature at the surface is higher than the deep water temperature due to solar heating and thermal convection in the water which keeps it that way since the heavier cold water remains in the depths and the warmer, less dense water stays on the surface.
- Atmosphere - The thin layer of gases above the Earth extends to about 800 kilometres deep with a temperature of -273°C (absolute zero) at its outer limits. Most of the atmosphere (about 80%) is actually within 16 km of the surface of the Earth. In, scale this would be equivalent to a generous coat of varnish on a desktop globe.
Geothermal Gradient
The geothermal or temperature gradient is the rate of increase in temperature per unit depth in the Earth due to the outflow of heat from the centre..
The temperature gradient between the centre of the Earth and the outer limits of the atmosphere averages about 1°C per kilometre. The temperature gradient in the Earth's fluid layers, the magma, tend to be lower because the mobility of the molten rock tends to even out the temperature. This mobility however does not exist in the solid crust where temperature gradient is consequently much higher, typically between 25 °C and 30 °C per kilometre depending on the location and higher still in volcanic regions and along tectonic plate boundaries where seismic activity transports hot material to near the surface.
At depths of 10 kms in the Earth's crust therefore the temperature could be as high as 300 °C which makes practical energy capture possible.
Energy Harvesting
There are two main exploitable sources of geothermal energy. Hydrothermal systems, first demonstrated in 1904, used the naturally occurring hot water or steam trapped in or circulating through permeable rock, to drive steam powered electricity generators.
See History.
More recently, since 1970, technology has been developed to extract the heat from hot rock by artificially circulating water through the rock to produce super-heated water or steam to drive the generators.
For cost efficient electricity generation, suitable temperatures for hot water and steam range upwards from 120°C to 370°C. Such naturally occurring hydrothermal resources are not widely available and are found in only a few regions of the world where the Earth's crust is very thin, usually around the edges of the crustal tectonic plates. Geothermal electricity generating plants have been installed in over twenty countries with new installations planned in several more.
In shallow reservoirs or regions where the water or steam temperature may range between 21°C to 149°C and not be hot enough for efficient electricity generation, the hot water can be used directly for local heating applications.
Hydrothermal Systems - Geothermal Aquifers
Conventional hydrothermal systems make use of geothermal aquifers which are naturally occurring geological formations of permeable rock or unconsolidated sediment (gravel, sand, silt, or clay) in which water may accumulate, between layers of impermeable rock. Where these aquifers occur in fractured volcanic rocks where temperatures are relatively high near the surface or in non volcanic areas where the crustal heat flow is very high, the water temperature may be high enough to provide steam for powering a conventional prime mover driving an electricity generator.
The hot water can be extracted from these hydrothermal reservoirs using boreholes and, after the heat has been extracted, the cooled water is pumped back into the ground to maintain the water table and pressure. Energy from geothermal aquifers is not completely renewable since heat is usually extracted at a rate quicker than it is replenished by the surrounding rocks.
Hot Dry Rocks (HDR) Enhanced Geothermal Systems (EGS)
Hot rock systems extract energy from dry rocks with temperatures up to 1000°C deep in the Earth's crust, rather than from hydrothermal aquifers, but first the solid rock must be made permeable to allow the circulation of water into which the rocks give up some of their heat. Such Hot Dry Rock (HDR) systems need Enhanced Geothermal Systems (EGS) to extract the available energy and these involve much higher investments and exploration risks than extracting energy from naturally occurring hydrothermal reservoirs.
Like hydrothermal systems, practical HDR systems depend on particular natural geological formations. They need access to hot granite or similar rocks with temperatures of 250°C or more, maintained by the heat flow from the Earth's hot core and such high temperatures are normally found at depths of over 3 kms. The deeper the rock, the higher the temperature but current drilling technology limits the practical working depths to about 5 kms. The ideal geological formation also includes an insulating blanket of sedimentary rocks, particularly shales, siltstones and coal seams, on top of the hot granite which effectively entrap the heat from the granite preventing it from being dissipated. Water is used as the thermal fluid to get the heat out of the rock and to enable this, the solid granite must be broken up (fractured) to allow horizontal water flow through the hot rock layer, and equally important, to provide the largest possible surface area of the hot rock through which the heat can be transferred into the water.
The water circulation system needs at least two bore holes, an injection bore hole through which cold water is pumped at high pressure down into the hot rock layer and an extraction borehole through which the hot water is returned to the surface.
The fracturing of the hot rock is achieved by the injection of water from the surface under extremely high pressures. The water pressure forces open existing fractures in the hot rock, which do not completely close again when the water pressure is removed, creating a passage through the rock between the injection and extraction boreholes. This is not an easy process because the immense pressures due to the weight of the overlying rocks tends close up any gaps in the rock. Nevertheless this EGS hydro-fracturing stimulation technology is commonly used in the oil industry to improve flow rates by enhancing the permeabilities of the host rock.
Superheated water extracted from the hot rocks is pumped to the surface and used to power a conventional electricity generating plant.
The diagram below shows the main components of a geothermal power plant used to capture energy from hot dry rocks.
Hot Dry Rock System In operation, cold water is pumped at high pressure down into the very high temperature fractured hot rock where it becomes superheated as it passes through the rock on its way to the extraction borehole(s). The diagram shows a "binary system" in which hot water emerging from the borehole is directed through a heat exchanger and after giving up its heat the cooled water is recycled back down the injection borehole into the hot rock bed. The working fluid, a low boiling point liquid, circulating through the secondary circuit of the heat exchanger is vaporised by the heat extracted from the well water and used to drive the turbine.
The available heat flow is given by : q = Kt ΔT z Where q is the heat flow per square metre in W/m2 Kt is the thermal conductivity of the rock in W/m/°C ΔT is the temperature difference in degrees Centigrade z is the thickness of the hot rocks layer in metres. Thus ΔT/z is the temperature gradient
| Geothermal Energy Capture from Hot RocksDiagram Source - Australian National University (Modified by Geothermal Resources Ltd) |
The diagram below shows the temperature gradient in the Earth's crust at different locations.
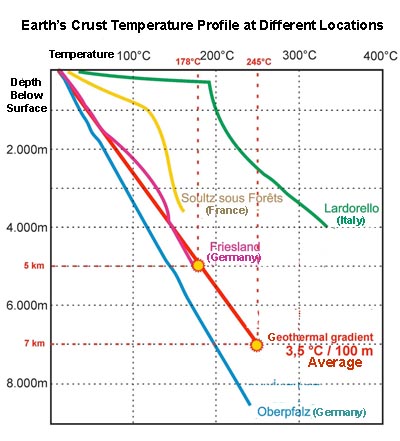
Source Geohil AG (Modified) | The temperature profile varies, depending on factors such as the porosity of the rock, the degree of liquid saturation of the rock and sediments, their thermal conductivity, their heat storage capacity and the vicinity of magma chambers or heated underground reservoirs of liquid. Low temperature gradients mean that boreholes must be very deep to reach high temperature rock but because of the difficulties involved in deep drilling and extraction, high temperature gradients are needed to get heat from reasonable depths. Suitable conditions are only available in a few locations
A constant heat flow can be obtained from hot rocks but the heated water extracted from the rock is often full of debris and pollutants.
Although the fuel is free and the maintenance costs are low, the exploration risks, the capital costs are very high. |
- Depletion
Geothermal energy sources are not strictly renewable if heat is extracted faster than it can be replaced by the heat flow emanating from the Earth's core. Over time, the hot rock source will cool as heat is continually extracted from it by the circulating water. Calculations indicate that in some locations it could take 30 years to cool and then 20 years to regenerate sufficient heat again. In an operating hot dry rock geothermal system, this would necessitate shifting of the circulation cell to another part of the hot dry rock body to allow time for heat regeneration. In this sense, hot dry rock geothermal energy is truly renewable and sustainable.
- Available Energy
The key factors influencing the amount of heat which can be extracted from a hot rock system are:
- The temperature of the hot rock
- The flow rate of water through the hot rocks
- The volume and surface area of the hot rock exposed to the circulating water
- The permeability in the hot rock (Sufficient to allow free flow of injected water through a large volume of rock)
- The energy needed to drive the water pumping system (Efficiency loss)
- EfficiencyThe thermal efficiency of ideal heat engines depends on temperature difference between input and output temperatures of the system.
Carnot's Law gives the maximum efficiency as (1 - Tc / Th) where T is the temperature in degrees Kelvin (See Steam Engine as a Heat Engine)
Hot Rock systems have a low efficiency because of low temperature difference between the water pumped out of the Earth and the water pumped back in. For a system with a water supply temperature of 85°C and a return temperature of 35°C, the maximum possible thermal efficiency will be
1 - (35+273)/(85+273) = 1 - 308/358 = 14%
Because of the energy lost in heat transfer from the rock and in the pumping energy required for the water circulation circuit, the system conversion efficiency will be much lower, possibly reducing the thermal efficiency by more than half in low temperature systems.
Ocean Thermal Energy Conversion (OTEC)
OTEC energy harvesting is similar to geothermal energy extraction described above except that the temperature gradient has an opposite slope. Sea water is heated by energy both from the Sun and from the Earth below. The solar energy falling on the water surface is greater than the heat flow emanating from the Earth so that the temperature at the surface is greater than the temperature in the depths of the water. OTEC systems convert the heat energy of the surface water into electrical energy using Binary Electric Generating Plants. (See below)
- Supply Characteristics
The available energy depends on the temperature gradient in the water.
Warm water at the surface vaporises a working fluid such as ammonia or butane and the vapour is used to drive a turbine generator. Cold water, pumped up from the depths (down to 1000 metres), condenses the vapour.
- Available PowerThe conversion efficiency depends on the temperature gradient in the water and is determined by Carnot's Law.
Only sites with at least a 20 degree temperature difference are likely to be economically viable. Unfortunately in most locations the temperature difference is much smaller than this.
For a 20 degree temperature difference the maximum theoretical maximum efficiency is given by:
1 - (5+273)/(25+273) =1 - 278/298 = 7%.
Practical installations will also have large pumping losses as well as heat losses within the system which reduce this efficiency even further and overall conversion efficiencies of 2% to 3% are typical.
Geothermal Energy Conversion Systems
Water, Steam and Other Circuits
The thermal energy available from geothermal sources is harvested and used in various forms and equipment for utility scale geothermal power generation is available in capacities from as low as 100 kW.
- Single Cycle Geothermal Power Plants
Generating electricity with conventional steam turbines requires working fluids at temperatures of at least 150 °C. This is only possible with high temperature geothermal sources of steam or superheated water which can be flashed to steam. The diagram below shows some of the possible variants when high temperature water or steam is available.
If steam is available directly from an aquifer it can also be used directly, after filtering, to drive a turbine. Otherwise steam can be generated from superheated water ejected by the aquifer.
Turbines are available with either back-pressure (atmospheric) or condensing exhausts. Condensing systems are more efficient but atmospheric exhaust turbine systems are simpler and cheaper. In a condensing system the condensate is usually reinjected into the ground, not necessarily into the aquifer. In an atmospheric system exhaust steam may be released to the atmosphere or used for other heating applications. | |
Early geothermal systems made direct use of hot dry steam generated in hot aquifers and erupting from geysers with temperatures typically above 235 °C, piped directly to steam turbines. This is by far the cheapest method of generating electrical energy from geothermal resources. The largest plants have generating capacities of over 1000 MW. Unfortunately there are few suitable sources in the world and dry steam plants are rare.
- Flash Steam Systems
Although there are not many natural sources of hot dry steam, superheated water at high temperature and pressure is available from many more hot water aquifers as well as hot rock systems. The boiling point of a fluid increases as its pressure is increased and superheated water is liquid water under pressure at a temperature higher than the normal boiling point of 100 °C. When the pressure is reduced the water flashes to steam. Superheated water pumped from the ground at temperatures of 175 °C or more can be flashed to steam in a separator or flash tank to drive a turbine directly. Surplus water from the flash plant is reinjected into the ground.
The most common geothermal electricity generating plants are currently flash generators but most newly planned installations are expected to use binary systems.
- Binary Cycle Power Plants
In many geothermal and hydrothermal plants the water temperature and pressure are too low for driving a steam turbine efficiently if at all. Dual cycle "binary plants" have been developed to make more efficient use of lower temperature water in the range from 100 °C to 175 °C. In a binary plant the hot water circuit passing through the thermal source is separated from the closed loop working fluid circuit used in the turbine by a heat exchanger. The hot water gives up its heat in the heat exchanger to a working fluid with a low boiling point and high vapour pressure at low temperatures when compared to steam. The working fluid is typically an organic compound such as ammonia, butane, pentane or isopentane which circulates through the secondary side of the heat exchanger where it vaporises and the vapour is then used to rotate a turbine in a conventional Rankine cycle electricity generating plant. After the vaporised binary liquid has passed through, and given up its energy to, the turbine it is condensed and recycled for re-use through the heat exchanger.
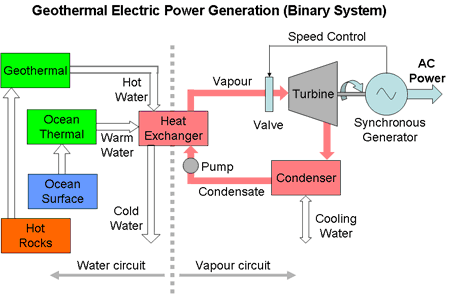
After passing through the heat exchanger the water in the primary circuit is pumped back down the bore hole into the hot rock. Binary systems have the added advantage that contaminated water from the primary heat source (salt water from the ocean or polluted water from aquifers and hot rocks) never comes in contact with the turbine generator units.
- Geothermal Systems
Super-heated water is brought from the hot rocks to a surface heat exchanger under pressure without boiling. By preventing the water from hot rock from boiling, this dual loop system also keeps the deposition of dissolved salts and other debris in the pipe systems to a minimum. The secondary vapour circuit is used for driving the turbine.
- Ocean Thermal Systems
The marine system is similar to the geothermal system adapted for the low temperature source. Hot water is taken from the surface of the ocean to vaporise the fluid in the turbine circuit. Cold water is pumped from the depths of the ocean to condense the working fluid.
See also the table of Energy Flows
See also Generators
Return to Electrical Energy Supply Overview
-
Geothermal Energy The Power From Below!
"Geothermal" is from two Greek words – "geo" meaning the earth and "therme" meaning heat. "Energy" stems from the Greek word "ergon" - meaning work. Literally translated, geothermal energy is "the work of earth's heat".So what is geo-energy...
-
Ocean Energy
What is Ocean Energy? All type of renewable energy which is acquired from the sea is called Ocean Energy. Constant flow of ocean currents contains huge amount of water across the earth's ocean. Technological development contributes to extract...
-
Geothermal Energy
What is Geothermal Energy? Basically, geothermal energy is the technique of gaining heat as energy source from earth’s surface. In order to produce electricity, most power plants require steam. Geothermal power plants use steam which is produced from...
-
Ocean Energy
The ocean can produce two types of energy: thermal energy from the sun's heat, and mechanical energy from the tides and waves. Oceans cover more than 70% of Earth's surface, making them the world's largest solar collectors. The sun's...
-
Heat And Internal Energy
Heat is defined as the transfer of energy across the boundary of a system due to a temperature difference between the system and its surroundings. When you heat a substance, you are transferring energy into it by placing it in contact with surroundings...
Green Energy